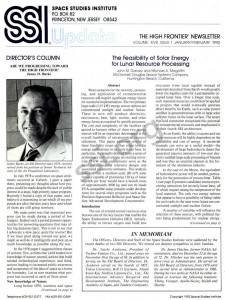
SPACE STUDIES INSTITUTE
P.O. BOX 82
PRINCETON, NEW JERSEY 08542
[[librarian note: This address is here, as it was in the original printed newsletter, for historical reasons. It is no longer the physical address of SSI. For contributions, please see this page]]
SSI UPDATE
THE HIGH FRONTIER® NEWSLETTER
VOLUME XVIII ISSUE 1 JANUARY/FEBRUARY 1992
DIRECTOR’S COLUMN
ARE WE PROGRESSING TOWARD
THE HIGH FRONTIER?
James D. Burke
In July 1978 a conference on space settlements occurred at Caltech. I gave a paper there, presenting my thoughts about how progress could be made despite the lack of public interest in a large, high-priority space program. Recently I found a copy of that paper, and I believe it is interesting to see which of my proposals are alive thirteen years later and which of them are still going nowhere.
My main point was that important progress can be made during a period of low budgets. Indeed such a period presents opportunities for answering questions that may affect big decisions later. This is not to say that I advocate a slow pace: quite the reverse! But if we must plod along toward our goal, we might as well do it intelligently and pick up as much knowledge as possible along the way.
In the 1978 paper I suggested three classes of actions: first, actions that yield needed new knowledge of nature; second, actions that build needed technological experience; and third, actions leading to increased public awareness and acceptance of the idea of space as a home for humanity. Let us now examine what progress has occurred in each of these areas.
New Knowledge of Nature
Long before 1978, scientists and space mission planners had reached agreement that any large and sustained attempt at off-Earth living must ultimately make use of off-Earth resources. Solar energy and the materials and environments of the Moon and the near-Earth asteroids are the resources of most immediate interest, while Martian resources may be important later. From its founding, SSI has led in advocating the discovery and use of extraterrestrial resources. To prospect for the material resources of the Moon, the right first mission is a remote-sensing polar orbiter instrumented to make global geochemical, geophysical and mineral maps. Many attempts have been made, both in the U.S. and elsewhere, to get this mission started but none has succeeded to date. In the absence of flight, mission scientists have done what they can from here, and improvements in telescopic spectrometry and image processing have yielded good maps, with resolutions of a few to a few tens of kilometers, for the distribution of some material on the Moon’s near side. Partial far-side coverage was obtained during the Galileo flyby of Moon and Earth in December 1990.
Meanwhile, studies of lunar samples from Apollo and Luna missions have continued, and the sample collection has been augmented by a few Antarctic meteorites now proven to have come from the Moon. New observations are not the only source of progress: Since 1978, theories of the Moon’s origin and evolution have advanced greatly. The leading hypothesis now is that a Mars-size body struck the Earth, throwing out material that coalesced into the Moon. Enormous heating events, clearly a part of the Moon’s legacy, correlate with the absence of water and other volatiles in lunar rocks. If there is any water, it may be in the form of ice in polar cold traps. Thinking about this possibility has recently been stimulated by ground-based radar observations that seem to indicate ice at the poles of Mercury.
Knowledge of lunar resources has thus advanced somewhat, but the definitive step is still in the future. Knowledge of the near-Earth asteroids is also improving, particularly in terms of discovering more of them, and the relationships among asteroids, comets, meteors, and meteorites are being actively debated and explored. Asteroid research is stimulated not only by scientific and resource considerations, but also by the growing recognition of the asteroid impact hazard to civilization on Earth.
Technological Advances
Finding natural resources is important, but so is learning how to use them. Extracting useful substances out of lunar raw materials has been among SSI’s research and advocacy objectives from the beginning, and it is most satisfying now to see that NASA and other organizations have recognized the need and are pursuing serious laboratory research. One leader in the field is the University of Arizona’s Space Engineering Research Center, where both lunar and Martian resource-processing concepts are actively explored. With the benefit of these lab experiments it will be possible intelligently to design pilot-scale and ultimately industrial-scale processes for use off Earth. Much remains to be done, but at least the ground-based part of the program that I hoped for in 1978 is now underway.
Public Education and Advocacy
My third proposal was founded on the idea that, if more people understood the central importance of extraterrestrial resources to our future in space, public policy would eventually become more favorable. I coupled this idea with the thought that the needed funding would be easier to obtain if the US acted in such a way as to reduce the threat levels in its strategic contest with the USSR. Happily, some progress toward that goal was in progress by the mid-eighties. Now, however, even though the Soviet military threat has crumbled, both their economy and ours seem unlikely to support bold new space initiatives in the near term. My last proposal dealt with that possibility: I urged that we educate and encourage the space leaders of the future, and that wish has been splendidly fulfilled with the founding, in 1987, of the International Space University. ISU and SSI share many aspects of a vision for our future in space, and I am very pleased to be able to participate in the activities of both.
Well, what did I suggest in 1978 that has not made much progress? Probably the most conspicuous subject is off-Earth agriculture. Despite the importance of intensive agriculture even here on Earth, NASA and other government sponsorship in the field remains quite limited. Some good research is occurring in both the US and Russia, but much more could be done. In February 1992 an international conference on life support and biospherics will go on at the University of Alabama in Huntsville. Small-scale NASA and Russian experiments will be reported there. The larger, privately-sponsored experiment, Biosphere II in Arizona, may point the way to needed further advances. Meanwhile, we will have to be patient and steady in our advocacy of this centrally-important part of a program leading to enjoyable and productive human residence on the Moon.
Editor’s Note: Each issue of SSI Update will feature an essay by an SSI Director or Governing Member. Professor Freeman Dyson will author the MARCH/APRIL column.
The Feasibility of Solar Energy for Lunar Resource Processing
John M. Garvey and Michael A Magoffin
McDonnell Douglas Space Systems Company
Huntington Beach, California
Abstract
Most scenarios for the recovery, processing, and application of extraterrestrial resources will require significant energy inputs for successful implementation. The two primary large-scale (>15 kW) energy source options are concentrated sunlight and nuclear fission. These in turn will produce electricity, microwaves, heat, light, motion, and other energy forms as required by specific processes. The cost and complexity of the facilities required to harness either of these two energy sources will be an important determinant in the overall feasibility of extraterrestrial resource utilization. This paper addresses several of these feasibility issues as they relate to solar energy applications in an early lunar base. In particular, the insights derived from a series of preliminary tests involving an existing terrestrial 75-kW solar concentrator serve as the basis for conceptual reference designs and associated parametric estimates. This analysis indicates that a medium-scale (80m*) solar furnace capable of processing 128 kg of lunar regolith per hour would have a launch mass of approximately 4500 kg and can be made EVA-compatible using concepts under development for several other programs such as the Precision Segmented Reflector and Space Station Advanced Development Concentrator.
Introduction
The use of extraterrestrial resources may become one of the key factors that enables the Space Exploration Initiative (SEI). Initially, the ability to extract oxygen and build new structures from local regolith instead of materials launched from Earth would greatly lower the logistics costs for a permanently occupied lunar base. Over a longer time scale, such material resources could then be applied to projects that would eventually generate direct benefits for Earth, such as solar power satellites in geosynchronous orbit or large solar collector farms on the lunar surface. The recent Stafford committee recognized this potential of extraterrestrial resources and emphasized it in their fourth SEI architecture.
As on Earth, the ability to access and use these resources will be highly dependent on the availability and cost of energy. A terrestrial example can serve as a useful model – the development of large hydroelectric dams that generated massive amounts of low-cost electricity enabled large-scale processing of bauxite and was thus an essential element in the formation of the aluminum industry.
In the case of the Moon, the equivalent of hydroelectric power will be needed, particularly for the generation of process heat. Table 1 presents candidate resource processing operations for an early lunar base, all of which require raising the temperature of the local material. The only two energy options that appear to have a possibility of being viable for such tasks in the near-term future are concentrated sunlight and nuclear fission.
A number of variables will influence the selection of these sources, with political factors being predominant for nuclear energy.
This paper addresses a technical subset of these issues as they apply to the viability of the solar option. A portion of the insights presented here were developed from ongoing company financed research projects that McDonell Douglas Space Systems Company (MDSSC) is conducting in cooperation with research partners that include Alcoa, the Space Systems Institute, University of Arizona, and Shimizu Corporation. Much of this research is centered on experiments with a 75-kW solar concentrator that MDSSC originally developed in the early 1980s as part of a terrestrial solar power program.
Whereas earlier papers have reported on initial experiments that are examining techniques for applying concentrated solar flux to simulated lunar material to produce a variety of products, the discussion here focuses on the higher level issues and requirements associated with assembling and operating the solar furnace that could provide such high fluxes on the lunar surface.
Operational Considerations
To define requirements for solar applications on the Moon, it is useful to divide processing operations into two basic categories fixed and mobile (Table 2). The tasks under the first entail the processing of large quantities of material(s), often continuously. As on Earth, the ore is delivered to a facility, then crushed, heated, processed, and melted or cooled, resulting in product and waste. These centralized tasks also tend to be those that are best suited for a large nuclear facility, although the opposite statement (that a nuclear plant is most appropriate) cannot be made without a case-by-case analysis that is outside the scope of the current discussion.
Several observations from the 75-kW solar concentrator tests are applicable to the design and operation of these fixed facilities. First, the receiver should itself remain fixed, independent of moving mirrors (referred to as heliostats). Otherwise, feed systems will be far more complex, and variations in the reflector orientation and sun angles could affect flux densities and thus overall processing (a major limitation with the existing 75-kW concentrator). Second, especially for high-temperature operations, concentrating sunlight downward directly into ore, as opposed to irradiating the receiver design. Furthermore, the potential then exists to explore placement of bushings and thermal valves at the bottom of the receiver to enable and control gravity-induced flow of the melt (a future MDSSC/SSI/Alcoa research subject). However, for medium-scale operations this direct irradiation approach tends to preclude consideration of the multi-heliostat/central tower plant configuration that was successfully demonstrated at the 10-MW Solar One facility.
Finally, although a two-mirror system is possible, three-mirror systems that still retain only one active heliostat can be achieved, thus reducing potential mechanism failures. Efficiency losses due to the extra reflecting surface are more that compensated by elimination of distortions in beam pattern and flux densities that can occur in the two-mirror system.
After assessing these operational issues, the decision was made to baseline the three-mirror system for this initial study, largely because of the superior beam control and reduced requirements for the receiver crucible. The resulting preliminary design concept is discussed at greater length later in this paper.
Mobile Operations
In contrast to the fixed facility, mobile operations on the Moon will generally be temporary and will involve a transformation of the local lunar environment. Thus, a mobile solar concentrator such as that proposed by Magoffin and Stone would melt the top several centimeters of regolith to create roads and stabilize landing pad surfaces, fracture large rocks to clear a construction area, or weld bricks and slabs of cast basalt together to create a new structure.
As with fixed facilities, there are several options for mobile concentrators based on the number of reflecting surfaces. The previously referenced mobile system depends on both a mobile concentrator and a semi-mobile heliostat. Alternatives include a single parabolic mirror with constant focus (comparable to the MDSSC 75kW Stirling Dish concentrator), or a reconfigurable, multifaceted mirror that enables great flexibility in beam-shaping. The fixed concentrator generally produces major focus distortions for any off-axis applications and appears to be impractical for performing trace patterns like those needed for welding. The multifaceted reflector is more complex but preferred here because of (1) the importance of beam-shaping for various applications and (2) an emphasis on minimizing the number of independent elements. Modern microprocessor technology combined with Digital Image Radiometer (DIR) techniques can enable highly accurate, near real-time position control of the individual facets. Additionally, such an adjustable reflector can function as the adjustable heliostat in the fixed solar furnace system.
For the following parameter estimates, it is assumed that this mobile reflector can be assembled using comparable truss structures and facets as in the fixed solar furnace. However, more refined estimates will eventually incorporate inputs for the drive motors, alignment sensors, and associated cabling as well.
Alternative Electromagnetic Energy Concepts
A number of other studies have addressed the potential for microwaves and other kinds of focused, non-solar electromagnetic energy to conduct some of the lunar resource processing operations mentioned earlier. While such alternative electromagnetic energy may be immediately superior to sunlight in terms of absorption and depth of penetration (particularly for extracting loosely bonded volatiles), there are significant variations in such regolith properties as dielectric value and mineral makeup that can prevent a standard comparison. Multiple phases induced by melting further alters the energy transfer mechanisms. Additionally, at the system level, energy conversion losses will necessitate a much larger energy source/collection area than would a direct solar concentrator, to the extent that they are no longer feasible. As an example, to irradiate a material with 25kW of 2.45GHz continuous wave energy (the maximum level applied in the Bureau of Mines tests), at least a 75-kW collector is required (assuming 33% efficiency for a Stirling receiver to convert sunlight into electricity and a subsequent 100% efficiency in the generation of the microwave radiation). The scaling factor is even worse if photovoltaic devices are used for the initial electricity generation.
Given these system losses and overall broader application potential for the directly applied concentrated solar flux technology, we have concluded that concentrated sunlight is at least one, if not the only, preferred electromagnetic heating technique.
Conceptual Design for a Fixed Solar Furnace
The following concept for a fixed solar facility on the lunar surface takes into account the operational issues discussed above, as well as data from parallel studies on space-based reflectors such as the Precision Segmented Reflector (PSR) and Space Station Advanced Development Concentrator. While such point designs are necessary for developing preliminary rough-order-of-magnitude (ROM) estimates of size, mass, and related variables, they are also useful because they help identify alternative technology options for creating such facilities (e.g., deployable versus erectable parabolic reflectors).
Figure 2 presents a design concept for a solar furnace facility that can collect and concentrate enough sunlight to melt 128kg of typical lunar basalt per hour. The three-mirror configuration was chosen because it requires only one moving surface. The University of Arizona currently has a small-scale version of such a device operating in Tucson. Using a 1.5-m-diameter parabolic concentrator, researchers there have successfully melted tungsten, which has a melt temperature of 3380°C.
In addition to the higher, steady solar flux on the lunar surface, there are several other benefits in comparison with terrestrial solar facilities. First, the 1/6g gravity enables a far lighter support structure. Second, the absence of wind loading and associated debris also simplifies structure design and enables the elimination of walls. Finally, the slower rotation rate of the Moon translates into slower tracking requirements/lower torques for the heliostat actuators. The primary disadvantages are (a) much larger thermal cycles, which can be accommodated by use of low CTE composites, and (b) vacuum/dust impact on the actuators. It should also be noted that the tracking and stability control requirements for the solar facility are far less demanding than that needed for PSR.
Reflecting Surface Area Estimation
The size of the parabolic mirror in Figure 2 is derived from the 1-ring/7 panel type B truss configuration. Using a 4.2-m-diameter panel (the maximum that can fit in the shuttle payload bay), this selection results in a parabolic surface with an effective collector area of slightly less than 80 m2 .
Optimally, the second reflecting surface the rigid, flat mirror in Figure 2 – would have an area 1.4 times that of the parabolic reflector to compensate for the loss ineffective area due to the 45-deg angular offset. This increased area would be manifested in the direction parallel to incoming sunlight. However, the parametric estimates involve symmetrical ring structures. Consequently, a 15.4-m-diameter, 2-ring/19-panel truss configuration is used here. This decision provides an extra level of conservativeness in the extrapolations made further on. lt also enables the application of this design to the heliostat, which in general would be larger than the fixed mirror to compensate for cosine terms associated with the sun’s daily and seasonal movements and the lunar latitude of the solar furnace.
With these baselined designs, it is possible to calculate a ROM energy throughput. In contrast to a terrestrial concentrator, where atmospheric distortion losses can limit peak midday flux values to well under 1000W/m2, the heliostat on the Moon will continuously receive an average solar flux of 1353W/m2 throughout the entire lunar day (there is a ±3% variation over the year due to the eccentricity of the Earth’s heliocentric orbit). Consequently, the effective collection area of the heliostat receives 80m2 X 1353 W/m2 = 108.2 kW.
For this study, a 10% absorption loss is used for each reflector. Again, this is a conservative factor because more reflective (but also more expensive) coatings are available. Also, losses in some spectral bands (such as UV) are not propagated evenly through all three mirrors. Given this value, the resulting available concentrated solar flux from the parabolic reflector is therefore 78.9 kW, after accounting for a 25% loss due to reflection off the basalt. This enables the melting of 128 kg of lunar basalt per hour.*
*For basalt, CP = 0.3 cal/° g, CF = 100 cal/g, TM = 1100°C, Ti = 100°C
Mass Estimate
Using the parametric relationships for PSR-type structures results in Table 3.
While this is obviously a first-order estimate, it provides a loose upper bound on mass projections because of the conservative factors noted previously. Furthermore, it indicates that the furnace can process its own mass in 35 hours. Thus, even with the large uncertainties in the extrapolated values, it appears that a solar furnace can begin paying for itself in a fairly short time span, less than one lunar day.
EVA Assembly Time Requirements
Using an estimate of 10 minutes of EVA time for assembling one panel and associated struts, it appears that 70 minutes should be sufficient for putting together the parabolic concentrator, while 190 minutes will be needed for both the heliostat and fixed reflector. Thus, two EVA days could be adequate for final assembly and integration of the solar furnace. While telerobotics may be of some use at this stage, another candidate operation for such technology would be site preparation and material layouts.
Most studies of reflector assembly have assumed on-orbit operations Solar furnace related EVA operations in l/6 g will require updating these assessments. McDonnell Douglas and NASA Langley are preparing to simulate a Precision Segmented Reflector Assembly operation in the company’s Underwater Test Facility. If done on the Moon, the axis of rotation about the support fixture might be realigned so that it is parallel with the gravity field and the astronauts.
Next Steps
The first refinement of the lunar-based solar furnace concept should involve an iteration of the structural design and eventually a Finite Element Model. In particular, the pointing and stability requirements for PSR structures are far more severe than those expected for non-imaging surfaces like those in the solar furnace (because of these requirements, the PSR structure should be able to function in the lunar gravity field) . Secondly, the heliostat concept could be updated with information concerning actuators, Sun tracking elements, and control systems.
If such a facility is to eventually become part of SEI, hardware testing of the assembly concepts should take place. The PSR simulations could serve as a model for implementing such plans. In parallel, application testing could proceed with candidate receiver/crucible systems.
Summary
In contrast to nuclear power, solar energy for both terrestrial and space applications has a benign, positive public image. The modularity and flexibility to perform a variety of operations are key reasons for considering its use in early lunar resource processing. The preliminary assessment discussed here, utilizing observations from ongoing solar concentrator tests and data from Precision Segmented Reflector studies, indicates that a medium scale system can be implemented for relatively small costs in terms of mass and lunar EVA. Furthermore, such a facility will have a fairly short payback time, as defined by the mass of processed lunar material compared with the furnace’s initial launch mass.
This paper was presented at the 1991/AF Conference and is reprinted with the permission of the IAF.
IN MEMORIAM
The Officers, Directors and Staff of the Space Studies Institute were saddened by the recent deaths of two SSI Directors. We extend our deepest sympathies to their families.
Dr. Yaichi Ayukawa, President of Techno-Venture Co., Ltd., passed away on November 30 at the age of 68. In addition to serving on the SSI Board of Directors, Dr. Ayukawa served on the boards of MIT, Tokyo University, B-R 31 Icecream, Nissan Kasei Kai, Nichiyu Lipsome Co., Ltd., The International Advance Research and Development Institute, The Engineering Academy of Japan, and Tandem Computers.
Dr. James Fletcher, former NASA Administrator, died on December 22 at the age of 72. Dr. Fletcher was the only person to serve twice as Administrator. He served on the SSI Board of Directors from 1979 until his second term as Administrator in 1986. During his two terms at NASA he either initiated or oversaw the following missions: Viking, Voyager, Apollo-Soyuz, Skylab and Shuttle.
VICE PRESIDENT’S COLUMN
Solar System Surprises
1991 ended with more tantalizing hints about the bounty of our solar system. In November, Dr. Duane Muhleman, professor of planetary science at Caltech, released radar photographs of the planet Mercury which indicate that its north polar region appears to be covered with a vast sheet of water ice. The New York Times covered the story on November 7 noting that the discovery suggests that the Moon may have similar polar ice deposits which would have exciting implications for space exploration.
Another very interesting and somewhat mysterious development was the discovery of a tiny object designated 1991 VG. This object has an orbital period virtually identical to that of the Earth and made a close approach to our planet in early December. The mystery surrounding 1991 VG has to do with whether it is a natural object such as an asteroid or a remnant of the Apollo program in the form of a spent rocket stage. If it is an asteroid, it’s a tiny one with a mass of less than 1000 metric tons. (Such a mass has a “street value” of about $10 Billion in LEO at current launch costs.)
According to Dr. Tom Gehrels of the Spacewatch Camera Project, the delta-v [change of velocity] required to match orbits with 1991 VG is only 600 meters per second from the Earth’s own orbital velocity. Dr. Gehrels asked me to thank the Space Studies Institute family for its crucial support of the Spacewatch Camera. He said that the project would not have gone forward without your help. The .91 meter telescope and ChargeCoupled Device array has been the source of literally hundreds of asteroid discoveries. We can all take great pride in the success of this wonderful new tool for asteroid detection.
Closer to home was another pleasant surprise with important ramifications for opening the High Frontier. In response to recent Presidential commissions, NASA appointed Dr. Michael D. Griffen as the agency’s first Associate Administrator for Exploration. Griffen announced his plans to concentrate on lunar exploration with a series of small-scale orbiting probes to be followed by robotic and piloted landers. In a recent article in Space News he cited SSI’s Lunar Prospector as a model for small lunar orbiter design.
Dr. Griffen’s interviews and speeches have generated speculation that NASA will procure one or more lunar probes in a commercial manner, as opposed to its traditional method of designing space hardware and letting bids for the construction of NASA’s own specific design.
In addition to several small orbiters, Dr. Griffen’s office is supporting the development of a spacecraft called Artemis or the Common Lunar Lander. This system would be used to deliver 200 kilogram (440 lb.) payloads to the lunar surface. Payloads could include lunar material processing, soil excavation tests or small rovers. The use of this system for lunar sample return missions is also under examination.
SSI is particularly delighted with these very practical suggestions for lunar exploration. As you may recall, we convened our own Lunar Systems Study in January of 1988 to look at ways to bootstrap lunar development. Our group recommended the use of a Surveyor sized lander for precisely the same sort of missions presently being discussed by NASA. Eagle Engineering sponsored the participation of premier space artist Pat Rawlings in our 1988 project. Pat, now at SAIC, has also produced the concept drawings for Artemis. The accompanying sketches show both projects.
It’s too early co know whether Dr. Griffen’s refreshing plans to concentrate on near term hardware projects rather than additional “architecture” studies will get the funding support required to go forward. However, it is good to see this practical approach to space exploration taking root within NASA. Griffen and his programs deserve our support and that of Congress. SSI’s research, made possible by your help, is once again providing a basis for space exploration planning.
Gregg Maryniak
Automated Fabrication in Space
by Marshall Burns
Marshall Burns is the president of Ennex Technology Marketing, Inc., a research, development and marketing organization in Los Angeles, California. He is the editor and co-author of the first book to be published on automated fabrication, “Rapid Prototyping: System Selection and Implementation Guide” (Management Roundtable, Boston, 1991). Readers interested in more information can contact Dr. Burns at xxx-xxx-xxxx.
When the European settlers came to America, they brought their hammers, axes, saws and barrels full of nails. With these tools and materials they built cabins, barns and forts. They did not bring the most important construction material they would need because they knew they would find plenty of timber at their new homesite. In fact, in many cases they had to get to work cutting down trees not only to provide lumber for construction but also to provide clear land to build on.
When we go to the Moon, Mars and the Asteroids, we know we will not find any trees or wood. But we will find plenty of other construction and industrial materials from which we can build shelters, factories and machinery, such as iron, aluminum and magnesium. The presence of these materials could save us the expense of launching steel beams and aluminum habitat shells, except for one major problem. What will stand as the modern analog of the settlers’ hammers, axes and saws? What tools can we take with us to turn celestial rocks and dust into walls and girders?
Until recently this was a “catch-22” situation. We need refineries and foundries to process raw ore into building materials. But refineries and foundries are large, heavy facilities that consume voluminous materials in their own construction. So how can a Lunar or other celestial settlement be bootstrapped out of the indigenous soil?
The answer may lie in a family of automated fabrication (AutoFab) technologies just emerging from laboratories around the world into immediate, Earthbound, industrial applications. These new machines take the data from a 3d CAD file describing the shape of a single, solid object and they make it! Typically, they build the object up one particle at a time, where the particle size is on the order of a few thousandths of an inch (about 1/10 of a millimeter). This is similar to the operation of a laser printer, composing its images on paper one dot at a time, but without the printer’s restriction to the 2-dimensional paper surface. By analogy to the laser printer’s “desktop publishing,” the new 3d processes are sometimes said to perform desktop manufacturing.
Another name used for these processes is rapid prototyping because of the first major application that they are being put to. Ford, GM and Chrysler all use these new machines to fabricate prototype designs for new or improved car components. Each company has collected dozens of stories of dramatic savings achieved by using rapid prototyping in place of the standard method of machining the parts on mills and lathes. The models can be output from the new machines overnight after an engineer has finished the design on his CAD station, instead of sitting in a queue for weeks waiting to be made up in the machine shop.
Prototyping has been an excellent first application for these still immature fabrication processes. A prototype often does not have to satisfy the same stringent specifications as the final item it is modeling. It may be needed only to check the design for “form” and “fit,” and not for “function.” But as the output quality of these automated fabrication processes improves, we are gradually moving toward a time when these machines will be able to make actual parts to be incorporated into working machinery.
The Processes
There is one automated fabrication process that is already a commercial success. It is StereoLithography, the brainchild of Chuck Hull and the core technology of a company called 3D Systems in Valencia, California. Unfortunately for space enthusiasts, this process requires an organic resin for its operation. The resins it uses are photopolymers which solidify under the influence of particular wavelength of light. The 30 Systems machines are incredible to watch as they scan a tiny needle of laser light over a vat of gooey resin, selectively curing the liquid into arbitrary shapes of plastic.
Another promising process, soon to be available on the market, is one that could use celestial materials. Selective Laser Sintering was invented by Carl Deckard while still a graduate student at the University of Texas at Austin. The machines are now built by DTM Corp., a B.F. Goodrich subsidiary formed for this purpose. This process scans layers of fine powder with a laser to melt and re-solidify the material into the desired shape. The powder can be almost anything: plastic, wax, metals. The metals are most interesting, and their use is still under laboratory development, but the machine has so far generated very sturdy, solid objects in plastic and wax.
Several other processes are available or soon to be introduced to market. Some of these use photopolymers as in StereoLithography. Others use thin filaments of material that are robotically guided into shapes and heated to the point of fusing into a permanent structure. Another takes adhesive-treated sheets of paper or plastic and, laying them down sequentially, laser cuts them to form the layers of the desired object, immediately stacking the cut shapes to build up the object.
Other processes are under development. A powder-based machine invented at MIT sprays adhesive on the powder to selectively bind it into the required shape. Another process guides a stream of molten plastic or metal to solidify in just the right combination of places to make a particular shape. Carnegie Mellon University is working on a method to build up metal objects by spraying the molten metal through a mask, like spray-painting through a pencil.
Off on the horizon, one can see these technologies being refined in accuracy to become the kinds of machines that Eric Drexler has been prophesying for nanotechnology, machines that build things, anything, one atom or one molecule at a time. When the methods are advanced to that level, the form of the raw materials available will be unimportant, only their atomic content will matter because the machines will be capable of decomposing the feed material into its constituent atoms and regenerating any conceivable object from them. This will not only be the ultimate style of manufacturing, it will also solve our recycling problems.
These ultimate machines are a long way off. But we don’t have to wait that long to use automated fabrication in space.
Space Buildings from Dust
Carl Deckard wrote in his patent, filed in October, 1986, that “the apparatus… may be useful where size of production facilities is a major constraint, such as on a ship or in outer space.”
The main problem with using Deckard’s laser sintering machines in space is that currently they take specially prepared powders as input. This is analogous to the old thermal computer printers that needed specially treated papers to print on. This is no good because what we want is to use materials that are readily available without much processing. It would be worthwhile for DTM Corp. to experiment with applying their process directly to Lunar dust to see if items of structural strength can be produced. Another possibility has been suggested by Hubert Davis of Davis Aerospace in Canyon Lake, Texas, who points out that one of the byproducts of ilmenite reduction is particulate iron. This may become a useful input material for the laser sintering process.
It is not necessary that the AutoFab machines work on completely raw celestial material. The European settlers did bring barrelsful of nails to assist in the construction of their homes from native timber. A workable process could require the processing of rocks or dust with a chemical agent that is brought for this purpose. As long as the chemicals needed are several orders of magnitude less massive than the quantity of materials they can be used to treat, the process that uses them will be a tremendous boon to space construction and manufacturing.
Automated fabrication is only a few years old on Earth and will undergo major refinements and improvements in the coming years. New methods will arise that present currently unforeseen possibilities for generating objects from celestially available materials. These new methods will allow explorers to venture forth with AutoFab machinery with which they will build their homesteads and their industrial facilities. While it has been recognized for some time that such machines would be necessary to really make space settlement feasible, companies like 30 Systems and DTM are finally bringing them into reality.
TRILOGY
By now you should have received your copy of the January/February issue of TRILOGY with Dr. O’Neill’s article beginning on page 47. Unfortunately, there was an error made in the editing process by the Trilogy team. On page 50, first column, 9th line under “The link between energy and industrialization” the line reads “less than five times the energy.” It was to read “is more than five times as frugal…” Dr. O’Neill has sent a letter to the editor, but we request that you clarify the point with anyone you share the article with.
In addition to supplying a complimentary copy to each SSI member, the publishers have offered to donate $4.95 from each subscription received from SSI members to SSI. We appreciate this offer and hope you will subscribe.
CORRECTION: The credits for the illustrations in the lower right of pages 1 and 3 of the November/December issue of “Update” were omitted in error. The illustration were done by Robert McCall and were supplied courtesy of Philip Friedman. They carry the copyright: Philip Friedman, 1991. We extend our apologies to both gentlemen.
©space studies institute